#Working3D: Six Questions for a University Professor Working on 3D Microstructures in the Biomedical Field
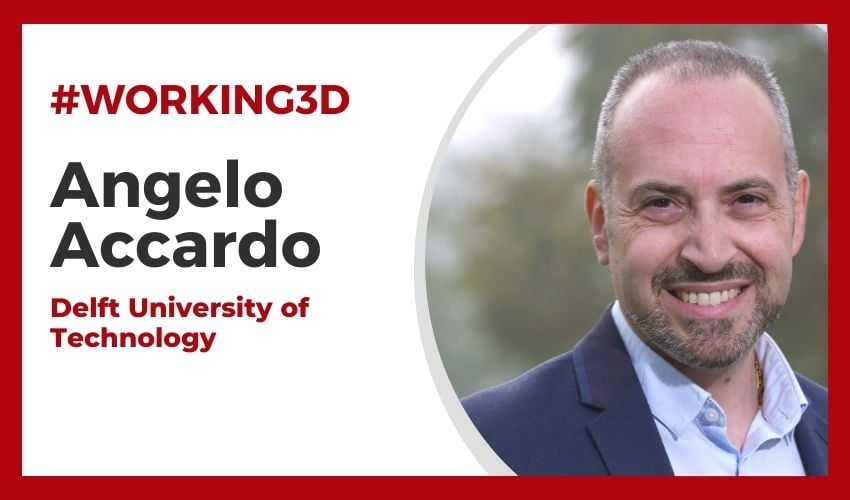
Advances in 3D printing and bioprinting in the field of medical research are increasingly promising. One of the uses of the technology is the development of 3D microstructures and nanostructures that can be used to understand how cells function in an environment similar to that of natural human tissue. 3D bioprinting is an excellent ally, for example, for 3D printing damaged tissue or tumors with human cells in order to increasingly accurately identify their function and identify effective personalized treatments.
Among the universities engaged in the research and use of 3D printing techniques for nanotechnology in the biomedical field is Delft University of Technology. To learn more about the research projects carried out by the university and what it means to work in this field and making 3D microstructures, we interviewed Angelo Accardo, Associate Professor in the Department of Precision and Microsystems Engineering at TU Delft.
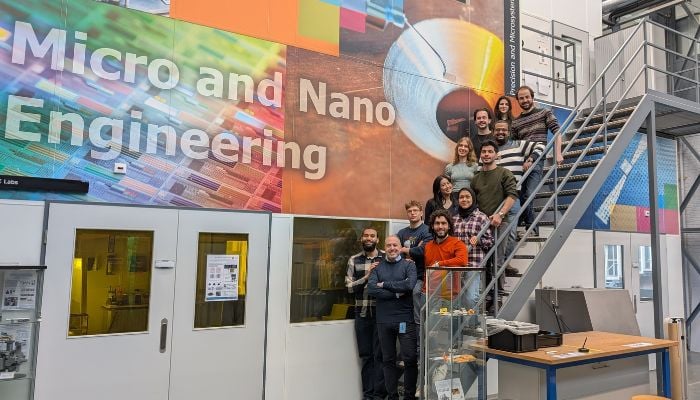
TU Delft team with Professor Angelo Accardo.
3DN: Could you introduce yourself?
My name is Angelo Accardo. I am an Associate Professor in the Department of Precision and Microsystems Engineering at Delft University of Technology (TU Delft) in the Netherlands.
3DN: How did you discover additive manufacturing?
During my post-doc at the LAAS-CNRS laboratory in Toulouse, France, I began to explore the use of light/laser-assisted additive manufacturing techniques. I worked particularly with stereolithography and two-photon lithography.
3DN: What are the major benefits and challenges of using 3D printing for nanotechnology and in the biomedical field?
These fabrication techniques make it possible to develop micro- and nanostructures with extremely high levels of resolution (up to 100 nanometers). At these scales, it is possible to create micro-scaffolds that can interface biomimically with healthy or diseased cells.
Such engineered microstructures can thus be employed to understand how cells function in an environment similar to that of natural human tissue. The next step will be to employ such 3D microstructures in tissue engineering and regenerative medicine, that is, for the regeneration of damaged tissue.
That being said, one of the remaining major challenges in this field will be to make such biomaterials completely biocompatible, in order to avoid adverse reactions of the remaining tissue. They also need to be biodegradable, that is to say “vanish” once regeneration of the tissue in question is achieved. In this regard, it will be necessary to employ scaffolds with relatively large overall dimensions (i.e. cm).
Two-photon fabrication technology is still relatively “slow” although extremely accurate. Therefore, it will be necessary to explore new approaches to increase the speed of fabrication, using, for example, two-photon “grayscale” lithography, or two-photon multibeam systems, to name a few.
3DN: Could you tell us about your current research on microstructures?
My research is based on the design and fabrication of 3D micostructures for three different types of applications: mechanobiology, in-vitro models of disease, and tissue engineering.
An article recently published on the cover of the journal Advanced Functional Materials, deals with the first case: 3D nanostructures fabricated by two-photon lithography have a diameter of a few hundred nanometers, resembling that of the fibers of the brain’s extracellular matrix, as well as that of filopodia (the “fingers” employed by cells to probe their surroundings). Their aspect ratio can be adjusted to provide a relatively low effective shear modulus (i.e., the elastic modulus detected by the cells when they crawl over the nanostructures) that approximates the softness of human brain tissue. These topographic and mechanical signals thus have a profound influence on the growth and directionality of neuronal networks.
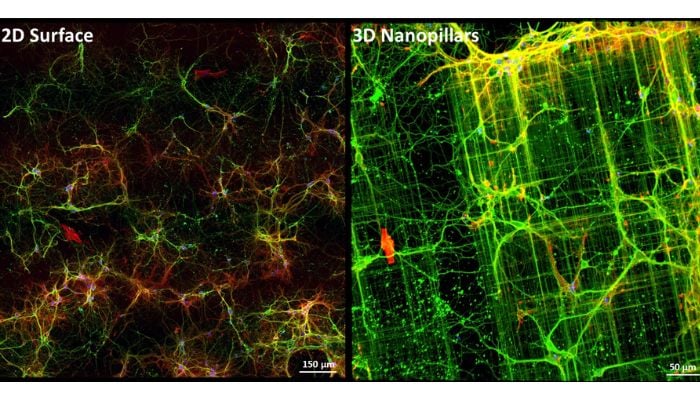
Comparison of neurons in 2D (left) and 3D (right) environment.
Compared with conventional “Petri dish” approaches, in which cells are cultured on flat, plastic or glass surfaces that are very rigid, our approach provides topographical and mechanical signals that are more physiologically relevant and greatly influence neuronal cell properties. For example, the directionality of the neuronal network and the morphology of growth cones (by which neurons probe the surrounding extracellular matrix to connect to other neurons) to name some examples.
Neuronal network development and growth cone morphology can be affected by neurodegenerative diseases such as Alzheimer’s disease (AD) and Parkinson’s disease (PD). With our approach, we demonstrated how it is possible to guide the formation of physiologically relevant neuronal networks and characterize growth cone morphology in a quantitative manner.
I envision the use of our platform as an in-vitro engineered disease model to understand the influence of major hallmarks of Alzheimer’s disease (amyloid plaques, neurofibrillary tangles) and Parkinson’s disease (Lewy bodies) on neuronal network directionality and growth cone development. We have also recently developed 3D models for the treatment of brain cancer (glioblastoma) by proton therapy, a treatment technique that, compared with X-rays (used in radiation therapy), uses protons, subatomic components, to target cancer cells.
We develop three-dimensional structures that mimic the microvascular system of the brain, where glioblastoma cells grow and proliferate. The goal is to provide mechanical, biochemical and geometric stimuli to the cells by mimicking the shapes of capillaries and blood vessels within the brain, through 3D structures that are made of biomaterial.
Once the glioblastoma cells are cultured in this biomimetic 3D environment, we bring them inside the Holland Proton Therapy Center, and expose them to different dosages of radiation. In this setup, it is possible to try to calibrate the proton radiation needed to damage the DNA of the cancer cells, and this without involving any animal model.
3DN: What qualifications and experience are required for someone in your position?
A basic engineering or physics education is helpful in developing a mindset suited to the scientific method. Nevertheless, during the years one learns countless aspects in addition to technological aspects, such as those related to cell biology and neuroscience.
To hold a faculty position in the Netherlands, one must also develop management and leadership skills that enable one not only to direct one’s own research group but also to fill strategic roles within the university.
3DN: What advice would you give to people hoping to work as a professor?
Passion, perseverance and patience. These characteristics are essential in academia and research along with the above. In addition, one must perpetually nurture one’s curiosity and be abreast of the current trends in order to write research projects that are then funded. Finally, it is essential to have a predisposition toward teaching in order to pass on not only notions but also an efficient modus operandi to the engineers and scientists of tomorrow.
What do you think of Angelo Accardo’s work on 3D microstructures at TU Delft? Let us know in a comment below or on our LinkedIn, Facebook, and Twitter pages! Don’t forget to sign up for our free weekly Newsletter here, the latest 3D printing news straight to your inbox! You can also find all our videos on our YouTube channel. You can also find more medical and dental news HERE.