Bioprinting Under the Microscope: Are the Current Standards Ethical?
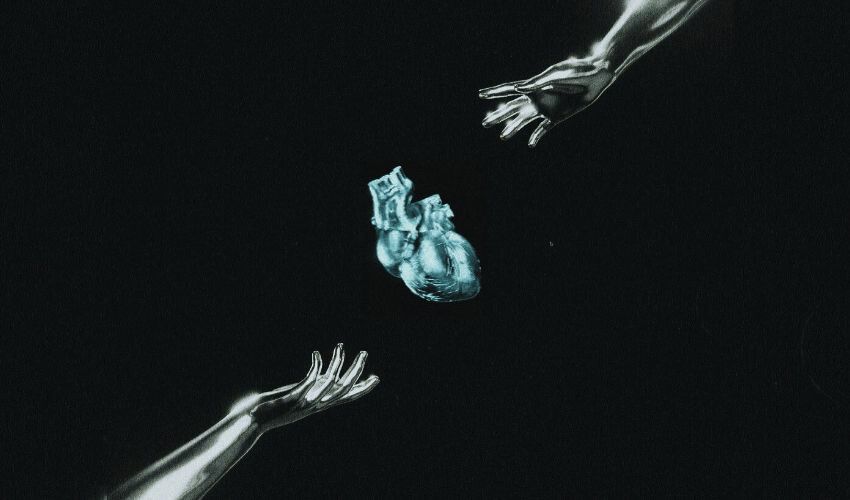
In the rapidly evolving landscape of biotechnology, three-dimensional (3D) bioprinting has emerged as a beacon of hope for transforming healthcare and addressing critical medical challenges such organ transplantation, tissue engineering, drug testing and development, personalized medicine, nerve regeneration, disease modeling, and much more. With the potential to revolutionize how we approach modern medicine, bioprinting is garnering increasing attention from researchers, investors, and healthcare professionals alike. As bioprinting rapidly evolves, however, it finds itself under the critical scrutiny of ethical considerations, legal frameworks, and regulatory challenges.
With continued growth attracting substantial investments, the need to confront and address the ethical complexities surrounding this revolutionary technology becomes ever more pressing. To better understand this, we took a look into the heart of the bioprinting industry, exploring the real ethical concerns that arise from the fabrication of artificial human organs, including the controversial use of human embryonic stem cells and the potential risks associated with induced pluripotent stem cells, digitization, clinical trials, and animal testing, among others.
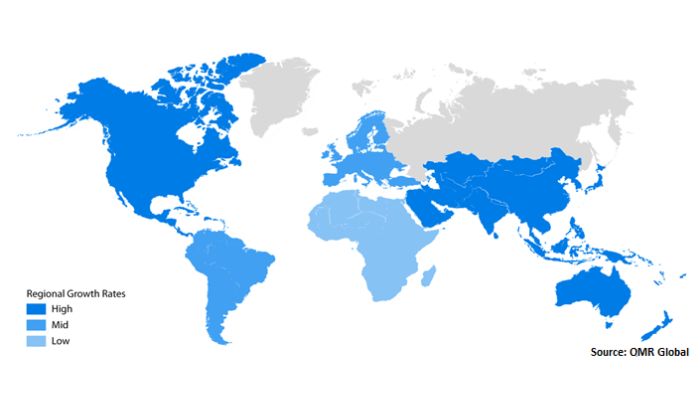
The global 3D bioprinting market size was accounted at 2.13 billion in 2022 and it is projected to hit around 8.3 billion by 2030 (Photo credit: OMR Global)
Putting bioprinting under the microscope, we’ll examine the intricate interplay between its extraordinary potential and the pressing need for responsible governance. Navigating these complexities, it is important to unravel the delicate balance between innovation and ethical accountability, thus ensuring that the future of medicine progresses with a clear conscience.
Universal Laws and Standards in Biomedicine
To comprehend potential improvements, it’s essential to delve into existing laws and standards. In the realm of biomedicine, these regulations form a comprehensive framework that oversees medical and biological aspects, research endeavors, and technological innovations. These laws and standards play a pivotal role in ensuring patient safety, addressing ethical considerations, upholding research integrity, and promoting responsible medical progress. This framework guides various domains, each with a distinct focus.
For example, Clinical Trials and Research Ethics take on foundational roles, overseeing the engagement of human subjects in research and clinical trials. Meanwhile bodies like Institutional Review Boards (IRBs) ensure informed consent, the protection of participant rights, and unwavering ethical adherence. Simultaneously, Government Regulatory Agencies such as the FDA shoulder the responsibility of supervising the entire trajectory of medical device creation. Data Privacy laws such as GDPR and HIPAA as well must not be forgetted as they are increasingly important as a way to protect the storage and use of sensitive medical data. In a similar vein, intellectual property must also be considered when it comes to innovations in the field.
One that is particularly important in the realm of bioprinting is Stem Cell Research which occupies a unique space at the intersection of scientific advancement and ethical consideration, outlining permissible boundaries that include the complex realm of embryonic stem cells. Meanwhile, Organ Transplantation navigates the delicate balance between patient well-being and the ethical sourcing of organs, fostering an equilibrium grounded in fairness and responsibility. And of course there are many more for various aspects in healthcare including reproduction.
Collectively, these legal dimensions create a landscape where biomedical progress is able to flourish. Adapting and evolving across diverse regions, laws and standards in biomedicine must stay calibrated to the ever-changing landscape of scientific progress and societal concerns. This dynamic equilibrium ensures that medical advancements are pursued within the boundaries of responsible and ethical conduct. In this way, bioprinting is not fully covered but is able to be pursued under the tapestry of legislation from different bodies.
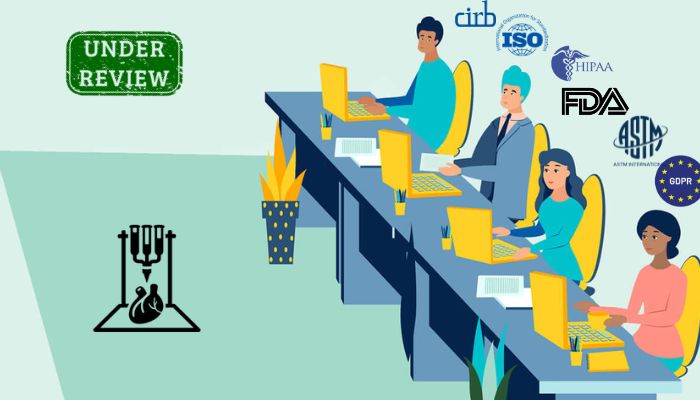
While currently there isn’t a comprehensive set of regulations that oversee the entire bioprinting process, there is partial legislation pertaining to tissue engineering and regenerative medicine (photo credit: Walden University)
The Laws and Standards Pertaining To Bioprinting
To further understand, it is now necessary to examine how the laws are applied directly to the ever-evolving field of bioprinting. Indeed, the section ‘Universal standards for tissue engineered products, in the study “Current standards and ethical landscape of engineered tissues—3D bioprinting perspective”, published by PubMed Central discusses the importance of universal standards for tissue engineered medical products (TEMPs) to ensure consistent quality worldwide. These standards are established by organizations like the International Organization of Standards (ISO) and the American Society for Testing and Materials (ASTM) International.
In fact, both ASTM and ISO have published a list of standards for TEMPs, covering various aspects like classification, biomaterials, cell and tissue constructs, safety, and assessments. These standards help regulate and ensure the safety and quality of tissue engineered products from pre-clinical research to clinical trials. Additionally, ISO has collaborated with various organizations and experts to continuously improve worldwide standards and quality, particularly for additive manufacturing techniques used in various fields, including medicine.
Overall, these universal standards play a crucial role in validating medical products, including tissue engineered products, and ensuring their safety and efficacy for human use. Since bioprinting is a rapidly evolving field, and standards and organizations involved in setting such standards, are continually developing. While specific standards may vary, there are general trends and aspects related to standards in bioprinting, which concern:
- Material Standards: There is a need for standardized biomaterials that are compatible with bioprinting processes and are safe for use with living cells. The materials used should have appropriate mechanical properties, biocompatibility, and degradation rates. Efforts are constantly underway to establish standards for the quality and characteristics of bioinks and scaffold materials.
- Cell Viability and Functionality: Standards are being developed to ensure that bioprinted constructs maintain cell viability and functionality. Bioprinting processes should not compromise the viability and behavior of the embedded cells, allowing them to grow, differentiate, and perform their intended functions.
- Printability and Resolution: Guidelines are being explored for the design and printing of complex structures. Printability factors, such as resolution, layer thickness, and nozzle diameter, need to be standardized to ensure the accuracy and reproducibility of bioprinted constructs.
- Bioreactor Culture: Bioprinted constructs often require post-printing cultivation in bioreactors. Standards are being considered for the culture conditions, including temperature, humidity, nutrient supply, and mechanical stimulation, to promote optimal cell growth and tissue development.
- Quality Control and Characterization: Standardized methods for quality control and characterization of bioprinted constructs are essential. This includes evaluating cell viability, cell distribution, tissue morphology, mechanical properties, and functionality.
- Ethical and Regulatory Considerations: Bioprinting involves working with living cells and tissues, raising ethical and regulatory considerations. Standards are being explored to ensure the responsible and safe use of bioprinting technologies, addressing issues related to patient safety, consent, and intellectual property.
- Bioprinter Calibration and Validation: Ensuring the accuracy and reproducibility of bioprinting processes requires standardized calibration and validation protocols for bioprinters. This helps achieve consistent results across different laboratories and systems.
- Data Reporting and Sharing: Standardized reporting of bioprinting processes, materials, and results is crucial for facilitating collaboration, reproducibility, and comparison of research outcomes. Open sharing of data and methods can accelerate advancements in the field.
Dissecting the Regulatory Landscape
Currently, there are significant challenges in the clinical transformation of engineered and bioprinted tissues (TEMPs). One major issue is the lack of standardized worldwide regulatory considerations for TEMPs, necessitating amendments in current standards and regulations. Regulatory guidelines vary across different regions, making it difficult to ensure consistent clinical translation of these issues. To overcome these challenges, many regulatory bodies adopt an adaptive approach in approving TEMPs, considering their complexity and non-reliability due to the use of natural biomaterials and cells. However, the scientific uncertainties surrounding TEMPs often result in delays in market approval.
Ethical concerns also arise regarding the choice of cell sources, with stem cells from adult donors being commonly used despite potential long-term safety risks and immunogenicity issues. Variability after implantation of TEMPs is another concern, as metabolically active cells in the host environment may cause immune reactions or mismatches.
Ethical Issues Surrounding Cell Use and Induced Pluripotent Stem Cells (iPSCs)
Adding to that, human embryonic stem cells (ESCs) have long been at the center of ethical controversies in bioprinting due to their derivation from human embryos. While they possess tremendous potential for differentiating into any cell type in the human body, their use raises profound ethical questions concerning the beginning of human life and the moral status of embryos. Obtaining human ESCs for research purposes involves the destruction of human embryos, leading to heated debates over when life begins and the moral implications of embryo destruction. Additionally, the commercialization of human ESC-derived products has been met with resistance from religious groups and ethical organizations. Religious considerations underscore the importance of engaging in transparent ethical discussions and considering diverse perspectives when developing guidelines and regulations for bioprinting involving human ESCs.
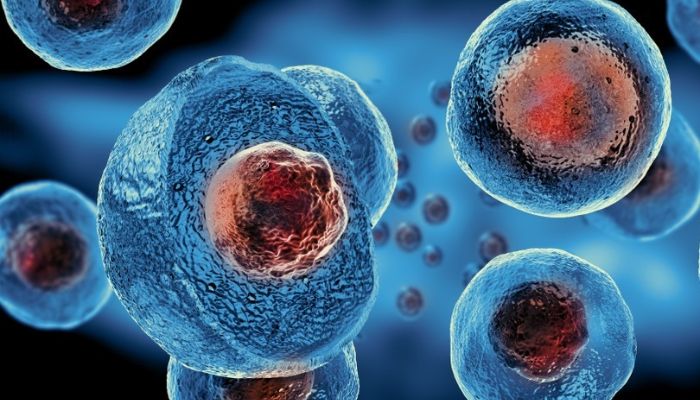
Embryonic stem cells (Photo credits: BioTechne)
Another ethical challenge arises from the use of induced pluripotent stem cells (iPSCs). The use of induced pluripotent stem cells (iPSCs) in 3D bioprinting holds immense promise for regenerative medicine. iPSCs are adult cells that have been genetically reprogrammed to possess embryonic stem cell-like properties, making them capable of differentiating into various cell types. This ability to generate patient-specific cells without the ethical concerns associated with human embryonic stem cells has made iPSCs an attractive option in the field of bioprinting. However, the usage of iPSCs is not without its ethical challenges. One significant concern is the potential for abnormal reprogramming during the iPSC induction process. Studies, such as the one conducted by Keisuke Okita, a lecturer at the Center for iPS cell Research and Application in Kyoto University, Japan, have highlighted the need for caution to avoid genetic aberrations and ensure the safety of bioprinted tissues or organs.
Moreover, tumorigenicity, or production of or tendecy to produce tumors, has been observed in iPSCs during stem cell therapy. A study by Andrew S. Lee, a researcher at the Institute for Stem Cell Biology and Regenerative Medicine, Stanford University School of Medicine, emphasizes the importance of addressing this risk to avoid the formation of tumors in patients receiving iPSC-based treatments. “Pluripotent stem cells (PSCs), including embryonic (ESCs) and induced pluripotent stem cells (iPSCs), offer immense potential as a source for regenerative therapies. However, the intrinsic qualities of self-renewal and pluripotency that make these cells so therapeutically promising are also responsible for an equally fundamental tumorigenic potential.”
The study goes on to mention that “the risks of PSC tumorigenicity have been highlighted over the past several years in a number of small and large animal studies”. Ensuring the safety and effectiveness of iPSC-derived products is vital to maintain public trust in the technology and protect patients’ well-being, and remains a hurdle for Pluripotent stem cell therapies.
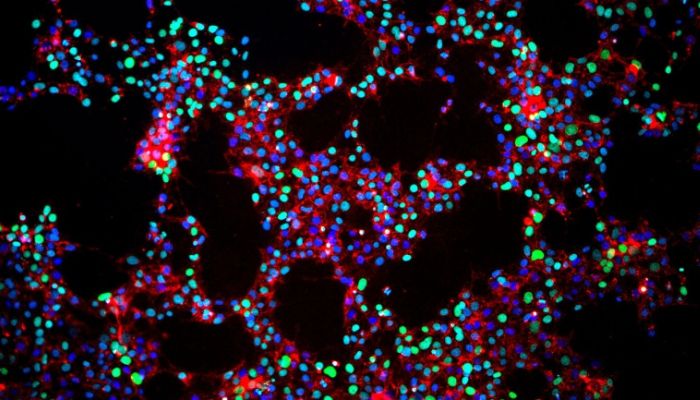
Microscopic view of Induced pluripotent stem cells (Photo credit: Gladstone Institute)
Ownership
Ownership of bio-printed products
Given that bio-printing involves the use of human stem cells and their genetic components, the question of who possesses ownership rights over the resultant bio-printed products is anticipated to fuel intense discussions. This debate extends to the realm of patient data and, in this context, the genetic material of the patients themselves. Parties with vested interests encompass healthcare providers, researchers, biotechnology firms, and, notably, the patients. To prevent the potential emergence of an illicit market for bio-engineered organs, it is probable that legal and medical experts will need to collaborate in order to establish clear ethical guidelines for managing these assets. This collaboration is aimed at ensuring fairness to both the patients and other involved stakeholders.
Ownership of intellectual property
Niki Vermeulen, a researcher at the Centre for Science and Technology Studies (CWTS), extensively explores the complexities entailed in formulating an intellectual property framework for the field of bioprinting. In a study on the socioethical view of printing human organs, Niki claims these “new forms of printing will have the same revolutionary and democratizing effect as book printing in their applicability to regenerative medicine and industry” predicting that biological structures designed for individuals will become “as available as text in modern literate societies.” She goes on to describe the similarities of process, development, accessibility between 21st century 3D bioprinting and the 15th century printing press, drawing several parallels.
The study poses a significant quandary revolving around determining whether bioprinting should be classified as a medical device eligible for patent protection or as a non-patentable medical procedure. This deliberation hinges on whether the technology should be regarded solely as a commercially viable innovation or as a fundamental medical practice for the future – possibly embodying both aspects. Moreover, an ongoing debate centers on whether the act of granting patents fosters or hampers innovation. Importantly, in this context, the outcome has the potential to exert a direct impact on the lives of millions. Vermeulen and colleagues suggest a plausible resolution to this predicament involves the collaborative engagement of both private and public sectors. This collective effort is aimed at establishing mechanisms for the creation and equitable distribution of research benefits.
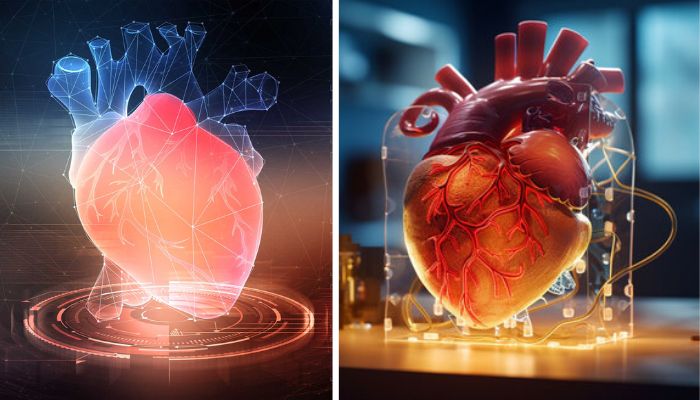
Ownership over one’s bio-printed and intellectual property is a future concern (photo credits: American College of Cardiology Foundation (left) and Pixstastock (right))
Clinical Trials
Designing Ethical Clinical Trials
Designing ethical clinical trials for 3D bioprinting becomes essential to ensure personalized and effective treatments. Inclusion criteria and the participation of terminally ill patients require careful ethical evaluation to balance potential risks and benefits, and to uphold ethical principles in research and clinical practice. The ethical and legal dimensions of 3D bioprinting are of utmost importance in the responsible development and application of this transformative technology. As we explore the vast potential of bioprinting to revolutionize healthcare, it is imperative to engage in ongoing discussions, implement robust regulations, and establish clear ethical guidelines to ensure its ethical and beneficial integration into mainstream healthcare practices. By addressing these ethical challenges proactively, we can pave the way for the ethical advancement of bioprinting, benefitting patients and society as a whole.
Looking to the future, 3D bioprinting holds promise, with potential commercialization of bioprinted tissue models and organ-on-chip within the next 5-8 years. However, commercialization of 3D bioprinted tissues and organs may take decades due to their complex nature and biological compositions. Overcoming challenges such as high-resolution printing, culturing heterogeneous tissues, developing vascular networks, and addressing ethical and safety concerns will be crucial to realizing the full potential of 3D bioprinting in clinical applications.
Are Current Practices Up to Standard?
But the real question is, are current bioprinting standards fulfilling their role? It seems that while current practices in bioprinting are progressing, they grapple with challenges in standardization and reliability. Despite significant advancements in bioprinting technology, there are instances where practices fall short of desired standards.
A significant hurdle pertains to the lack of standardized biomaterials suitable for bioprinting processes. Material property variations can lead to inconsistencies in mechanical properties, degradation rates, and biocompatibility of printed constructs, affecting overall functionality and safety. Another concern involves the viability and functionality of bioprinted constructs. While researchers strive for cell viability, instances arise where the printing process compromises embedded cell survival and behavior notably as a result of mechanical forces.
Furthermore, the lack of standardized protocols for characterizing and evaluating bioprinted constructs hampers quality control. Variations in assessment criteria make cross-study result comparison challenging. Another salient challenge concerns integrating functional vascular networks into bioprinted tissues for proper nutrient supply. Efforts to create functional blood vessels have faced complexity, impacting the vascularization of larger tissues and techniques must be improved. Furthermore, varying scenarios, such as generating human organs, raise concerns about organ ownership, patient consent, and regulatory pathways. This makes comprehensive ethical and regulatory frameworks specific to bioprinting increasingly necessary.
In short, while bioprinting has advanced, challenges with biomaterials, cell viability, and characterization highlight current practices falling short. These challenges emphasize the importance of ongoing efforts for standardized guidelines and protocols to enhance bioprinting’s reliability and consistency. These examples underscore bioprinting’s complexity and the need to address technical, ethical, and regulatory challenges for universally accepted standards.
Do you think the current standards are sufficient in the face of these ethical considerations? Let us know in a comment below or on our LinkedIn, Facebook, and Twitter pages! Don’t forget to sign up for our free weekly Newsletter here, the latest 3D printing news straight to your inbox! You can also find all our videos on our YouTube channel.
*Cover photo credits: Deep-image.ai
Bioprinting AM printing processes are very limited when looking at standard AM processes. I really don’t think any can be used. To open this article with the terms 3D printing is very misleading.
The very few readers of these discussions with a good understanding of 3D printing is not helpful.
Please consider simplifying the concepts and maybe more readers may comment.
When compared to conventional AM printing procedures, bioprinting AM printing techniques have very few applications. In all honesty, I don’t think any of these can be utilized.
There aren’t many uses for bioprinting AM printing processes compared to the more commonplace traditional methods. I really doubt any of these have any practical use.
I understand your concerns about the terminology and clarity in discussing bioprinting versus standard AM processes. It’s crucial that such articles are accessible and accurately represent the complexities of the technology to engage a broader audience effectively. Simplifying the concepts without compromising on the technical accuracy could indeed help more readers join the conversation. Thanks for pointing this out!